The role of gas and the gas infrastructure within the future energy system - a techno-economic assessment
Partners: ETHZ (FEN), ETHZ (PSL), TEP Energy, WWZ, VSG
Duration: 11/2017 - 07/2021
Funding: SFOE
Project Leader: FEN
Project Team: Dr. Adamantios Marinakis, Dr. Turhan Demiray, (Dr. Giovanni Beccuti till July 2020)
PSL Team: Prof. Gabriela Hug and Conor O Malley.
This project, focusing exclusively on the distribution and final consumption of energy carriers (i.e. bulk generation and transmission are out of the project scope), developed tools to model and quantify a coupled planning and operation of the electricity, gas and heating energy sectors (sector coupling). The results of this project assess to which extend sector coupling enables to better meet the challenges of the energy system of the future. At the same time, the followed "coupled" approach, allows to identify the potential value (or lack of value) of gas distribution infrastructure into the future energy system.
In this project, a set of potential future pathways are assessed in terms of their total cost for meeting the end energy demand, served by a local utility (WWZ), over a 40-year time horizon. The assessment is performed by means of an optimisation tool for multi-carrier system planning that was developed as part of the project. The total cost consists of: (i) customer investments in heating technologies (see below) and rooftop PV; (ii) utility investments in expansion of the medium voltage (MV) electricity network batteries, electrolyzers and fuel cells; and (iii) utility operational cost, i.e. the cost for the utility to buy electricity and gas from the wholesale.
The ongoing decommissioning of nuclear power plants and the increasing penetration of, often decentralized, intermittent renewable energy sources is progressively transforming the Swiss electric power system. Power flow patterns become more variable, while there is an increase of power flow from distribution to transmission. Wholesale electricity prices are expected to become more variable, as the value of electric power will be varying in a diurnal and seasonal manner, depending on the net demand (or excess power production) of electric power. Flexibility will be increasingly valuable.
At the same time, the Swiss strategy for the energy transition, together with technology economics, motivate an increasing electrification of the end demand, especially end demand for space heating. As a result, final electricity demand is expected to increase. Noteworthy is the fact that this increase, instead of being proportionally distributed throughout the year, is expected to have a seasonal sign. On the other hand, building efficiency improvements, as well as the fact that heat pumps draw the larger share of the heat that they produce directly from the environment are probably mitigating the increase in final electricity demand.
In the context of such an "electrification pathway", the role of distribution gas infrastructure in the future is questionable. It is speculated that too little final demand for gas will remain to justify the business of maintaining and operating the gas distribution network.
On the other hand, since in many Swiss regions the gas distribution network is anyway there, there is increasing interest in investigating whether this infrastructure can have a value in the future as a means to provide flexibility required by the electric power system. A so-called "coupled" planning and operation of the energy sectors of electricity, gas and heating might allow to increase the overall energy system economics and efficiency.
As a matter of fact, energy networks, such as electricity and gas grids, have traditionally been planned and operated independent from each other. This project, focusing exclusively at the level of distribution, developed tools to model and quantify such a coupling of energy sectors. The results of this project assess to which extend sector coupling enables to better meet the challenges of the energy system of the future. At the same time, the followed "coupled" approach, allows to identify the potential value (or not) of gas distribution infrastructure into the overall future energy system.
A four-step approach was followed:
- A set of future scenarios were defined, each corresponding to a different future pathway regarding the technologies utilised for heating (heat pumps, district heating, gas boilers and fuel cells), the electricity demand and the penetration of rooftop PV.
- Based on the defined scenarios, final energy demand for electricity and heat in the jurisdiction area of WWZ are determined in high temporal and spatial resolution based on a bottom-up future building stock model.
- To perform the overall analysis at the final stage, a modelling and optimisation tool for multicarrier system planning has been developed. Precisely, the approach followed in this project was to formulate and solve an optimisation problem which simultaneously considers:
(a) both energy carriers, i.e. electricity and gas,
(b) the cost for purchasing electricity and natural gas from the wholesale,
(c) the constraints imposed by the electricity distribution network,
(d) investments in new infrastructure, namely upgrade of the electricity distribution network and utility-scale batteries, electrolyzers and fuel cells.
- Finally, a techno-economic assessment of potential future options in electricity and gas distribution has been performed, considering a coupled planning and operation of WWZ network for given future demand, PV penetration, electricity and CO2 price, as well as technology cost scenarios.
In this project, the various potential future pathways are assessed in terms of their total cost for meeting the end energy demand, served by a local utility (WWZ), over a 40-year time horizon. The total cost consists of:
- customer investments in heating technologies (see below) and rooftop PV;
- utility investments in electricity network expansion, batteries, electrolyzers and fuel cells;
- utility operational cost, i.e. the cost for the utility to buy electricity and gas from the wholesale.
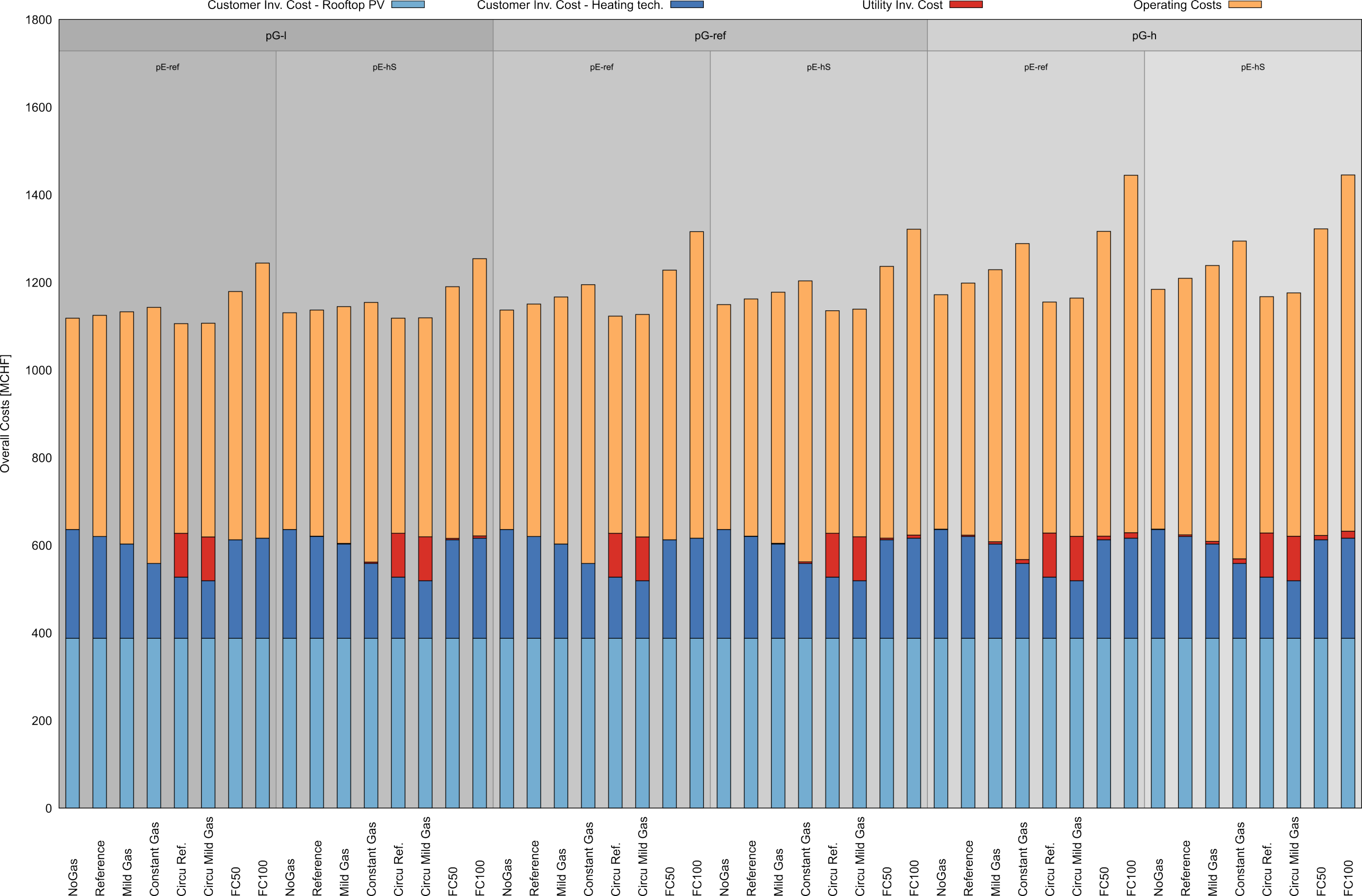
Key results
- The cost differences between a pathway of full electrification at distribution level (dominated by building-level heat pumps) and a pathway in which gas maintains a considerable role in serving the end demand for heating is relatively low (below 5 %, i.e. up to 50 million CHF of total cost over a 40-year time horizon) or even zero.
- In districts or regions where a convenient source of environmental heat is available (such as the Lake of Zug in this study), investing into a heat-pump-based district heating system seems to be the most economic option in the long-term.
- A pathway of full electrification (of customer space heating) is economically feasible.
- Policy decisions are required in order to prioritize a specific desired evolution, otherwise it is reasonable to assume that both a full electrification pathway and a pathway relying on gas for heating will develop to some extend in different regions.
- The MV electricity distribution networks considered in this study turned out to be capable of coping with an increased electrification path. Network upgrades can still be required in order to maintain a high degree of redundancy and, hence, reliability.
- In case of congestion, upgrading the electricity network infrastructure is more economic than resorting into alternative options such as batteries or sector coupling, which might have a role only if electricity network upgrade is impossible for other practical (not strictly economic) reasons.
- The tool co-optimizes two energy carriers: (i) electricity, and (ii) gas
- Multi-period optimization with utility-scale investment decisions: the length of interval between years can be chosen (5-year, 10-year intervals)
- Investments may consist in batteries, electrolysers, fuel cells, transformers, upgrade of network branches (e.g. add a new cable)
- Each year is modeled in hourly resolution
- Gas network is not represented explicitly but at the interfaces with the electric grid via electrolysers and fuel cells
- Electric grid is represented in DC
- The tool is based on Julia language.
- Input data:
- Infrastructure
- GIS
- Fuel/CO2 prices
- Technology costs
- Solar PV potential of buildings
- Heating demand of buildings
- Electricity demand of buildings
- PV penetration scenarios
- Scenarios on heating technologies and electricity demand